Composed by Scarlet Rivera “A musical journey connecting natural landscapes, climate, and biodiversity”. Stay tuned for the premiere!
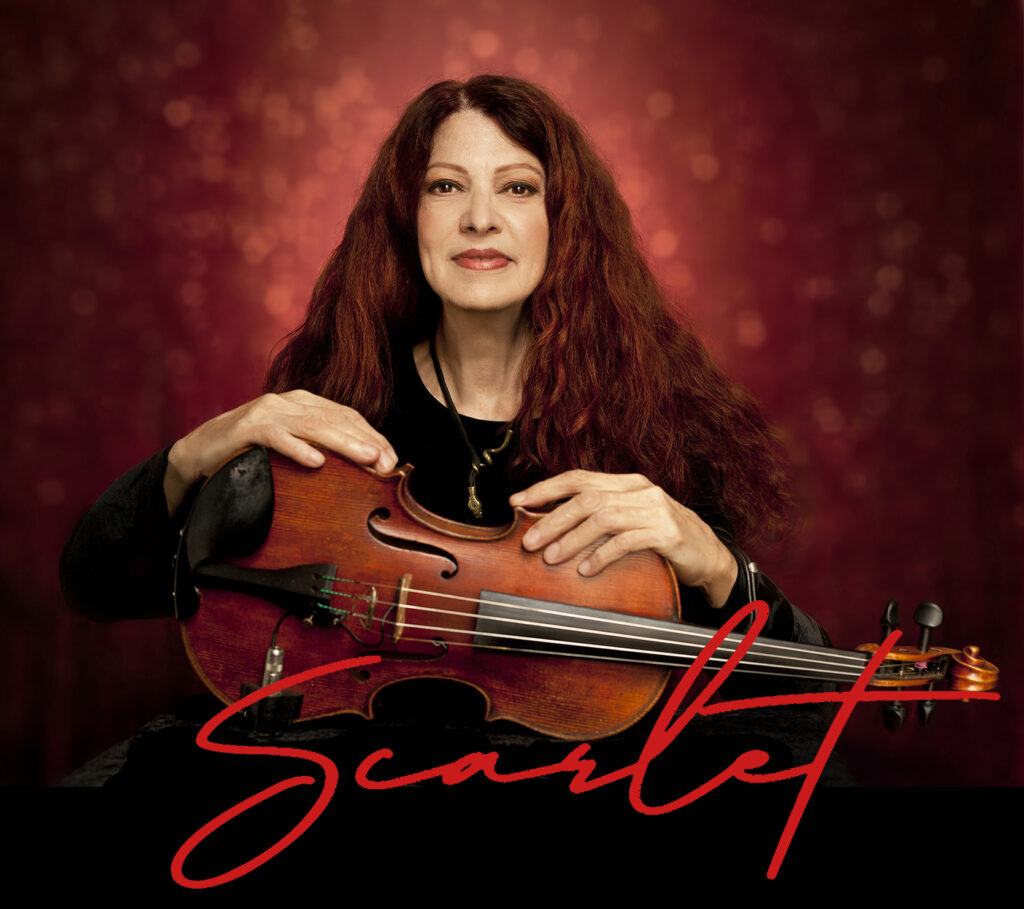
This is the intro video for “Voice of the Animals”. Music by Scarlet Rivera with Eduardo Del Signore. Visuals by Angelo Chiacchio. In collaboration with The ClimateMusic Project.
We are living in a garden with a billion diverse beings blooming in a diversity of form. A majestic cacophony of all living things sings the song of life. Even a grain of sand can sing the history of eons of life on Earth, beginning in the deep blue world: a mesmerizing intelligence in perpetual motion, the ocean. On land each living creature has meaning and purpose, every ant, bee and bird. The Earth itself is alive and has a voice.
I wrote the music of Voice of the Animals to honor all of nature’s voices. My music gives expression to these unique and irreplaceable beings. The science and sonifications reflected in the music confront the devastation of all that beauty, the trembling terror felt even by the trees of the threat of annihilation of their world.
From the world of tiny insects to the watery world of the pink river dolphin, to the mighty lion and elephant: They are calling us to reclaim our connection and to remember that we are not just spectators – we are the hope and promise for safeguarding the natural world into the distant future.
Scarlet Rivera, January 2024
Coming Soon!
Climate change, wildlands conservation, and maintaining the abundance and richness of life on Earth are often talked about in the media as if they are completely separate challenges. But in fact, they are linked in fundamental ways. Voice of the Animals highlights this inter-relationship. The section that follows is part of the science briefing that we provided to Scarlet Rivera at the beginning of this project. It was drafted by one of our science advisors, Dr. Garrett Boudinot.
I. Wildlands destruction: Causes and Consequences
Local land-use change such as deforestation has significant consequences for global climate. Deforestation can contribute directly to climate change by releasing the heat trapping greenhouse gas CO2, particularly when fire is used to clear trees and brush. The newly-uncovered ground after deforestation dries out more quickly (Malhi et al., 2008), erodes away more easily, and holds more heat (Shukla et al., 1990), all of which can further release greenhouse gases. In addition, deforestation reduces the capacity of forests to naturally reduce global warming by drawing down and storing CO2 in trees and soils. Because of these climate consequences, land-use management decisions at a local level can impact people and animals far away and far into the future.
Local climates (i.e., “microclimate”), including local precipitation, temperature, and humidity, are also directly impacted by deforestation. The removal of trees increases the temperature of the ground, reduces humidity at the ground level, and, when performed on a large scale, deforestation can even significantly reduce the amount of local precipitation (e.g., Shukla et al., 1990; Malhi et al., 2008).
Deforestation directly impacts local ecology. The removal of trees reduces habitat and food for wildlife species, from “microfauna” such as insects to “macrofauna” like apex predators. The altered microclimate from deforestation can change the habitability of the area for the animals and plants that live there. Furthermore, deforested land is often used for the grazing of cattle or the growing of monocultured crops (De Sy et al., 2019), which are managed by people who actively remove wildlife, and which also can further release greenhouse gases.
Because deforestation is often driven by agricultural expansion (De Sy et al., 2019), the history of deforestation follows a similar trend of increased human population for whom increased food production is needed (Fig. 1). The majority of deforested land is converted to cropland and pasture for crops and livestock, respectively, to feed growing populations. However, the specific post-deforestation land use is dependent on the geographic, ecological, environmental, and sociopolitical conditions of the cultures deforesting (De Sy et al., 2019). As a result, limiting deforestation is a complex objective that requires awareness of both cultural and environmental factors.
Habitat loss is the second leading contribution to carbon dioxide emissions and climate change, after the burning of fossil fuels. Connecting deforestation to climate change warrants nuance, and should invoke comparisons between local impacts of “global change” (e.g., reduced biodiversity, altered ecosystem health, land-use change, and agricultural expansion) and “climate change” (e.g., changes in global temperatures from greenhouse gas emissions). They are intertwined, and tell complementary stories.
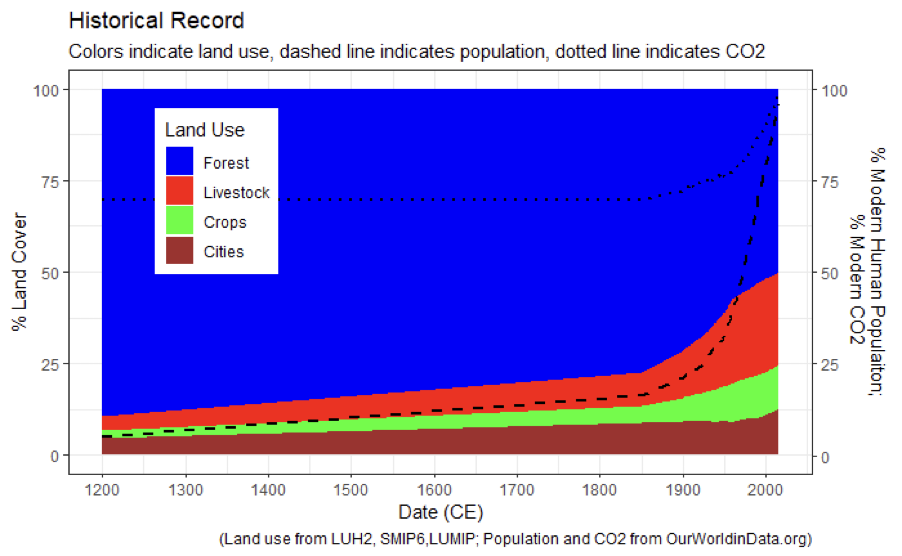
II. Responses to Deforestation: Reduction, Reforestation, and Afforestation
While limiting deforestation requires complex sociopolitical considerations, scientists agree that reducing deforestation is beneficial to global climate, local ecology, wildlife conservation, and even overall human wellbeing (e.g., Bradshaw et al., 2007; Newton and Benzeev 2018; Wolff et al., 2018). Reducing the rate and geographic extent of deforestation will help mitigate global climate change by reducing CO2 emissions, protecting the natural storage of CO2 in trees and soils, and maintaining heat-reflecting cloud cover (Malhi et al., 2008). But many scientists also recognize the potential for reforestation (e.g., actively planting trees and restoring previously-razed forests) to help fight climate change (e.g., Houghton et al., 2015). Reforestation can draw down and store CO2 in soils and trees, can further reduce heating solar rays from staying in the atmosphere, and can provide essential habitat for wildlife species otherwise threatened by climate change.
However, aside from the cultural considerations behind deforestation, there are several ecological challenges to reforestation. Some locations that had been covered by forest in the past may not easily be reforested because of soil degradation or microcliamte alteration that occurred since original deforestation (e.g., Shukla et al., 1990). Alternatively, changes in wildlife populations in some areas limit reforestation – large populations white tail deer in some parts of the US, for example, consume young trees faster than they can be planted and grow (http://www2.dnr.cornell.edu/ext/bmp/contents/nonharvest/non_damage.htm). Moreover, impacts of climate change are reducing the capacity of forests overall to benefit the climate; increased frequency and extent of forest fires make forests less capable of storing CO2 over long timescales (Dass et al., 2018). Thus, in some scenarios, deforestation represents one of the potentially irreversible impacts of climate and environmental change, highlighting the importance of reducing deforestation and climate change before further irreversible consequences emerge.
Because of changing climate and changing land-use, some scientists highlight the potential for afforestation, which establishes forests where previously no forests existed (Arora and Montenegro, 2011). Ideal afforestation candidates may be locations that had previously not been habitable to forests, but because of changing climate, are becoming more suitable for large stands of trees. However, again, any areas subject to reforestation or afforestation are areas currently used either for human habitation or food production, and thus sociopolitical and equity costs constitute major limitations to these as significant strategies to mitigate climate change currently.
III. Future Projections of Forests, People, and Land Use
Because deforestation and land use integrate both climate and societal factors, it is helpful to have social/environmental models to project how land-use and climate may play out in the future. An international team of climate and social scientists have developed such models to generate future projections based on current understandings of the climate, historical records of humans and land-use, and estimates for future demographic and climate shifts. Ultimately, the projections help assess the long-term impacts of policy decisions made today, and thus are included in the Assessment Report of the Intergovernmental Panel on Climate Change (IPCC) and in policies such as the Paris Agreement. Because a number of different decisions and social/environmental factors work together in different ways, scientists have imagined several “pathways” to inform the projections, which account for how socioeconomic decisions and demographics will play out – these pathways are thus called “Shared Socioeconomic Pathways,” or SSPs. Each of these SSPs project different global and regional changes in human behavior, land use, and climate.
There are 5 SSPs, ranging from “Sustainability” (SSP1) that mitigates climate change while advancing human equity, education, health, and well-being, to “Fossil-fueled Development” (SSP5), which exacerbates existing economic divides and goals through continued fossil fuel consumption while allowing some segments of the population to adapt to an altered climate state. SSPs also show different climate outcomes represented by global warming (radiative energy flux, in Watts/m2), with higher numbers indicating more global warming. SSP1 and 4 both project scenarios of climate change mitigation, though SSP1 accompanies low challenges to adaptation, while SSP4 accompanies high challenges to adaption. For simplicity, we focus on these two projections at two different warming potentials: SSP1 at 2.6 Watts/m2, and SSP4 at 3.4 Watts/m2.
Deforestation plays out very differently on global scales for these two projections (Figs. 2, 3). SSP1 shows significantly reduced deforestation in the future relative to today (Fig. 2), while SSP4 shows continued deforestation (Fig. 3). Thus, while both projections “mitigate” global climate change, they do so quite differently with respect to land use, which may contribute to their different final radiative energy. Notably, none of the SSPs show reforestation on a global scale, highlighting the functional irreversibility of the forests hitherto razed.

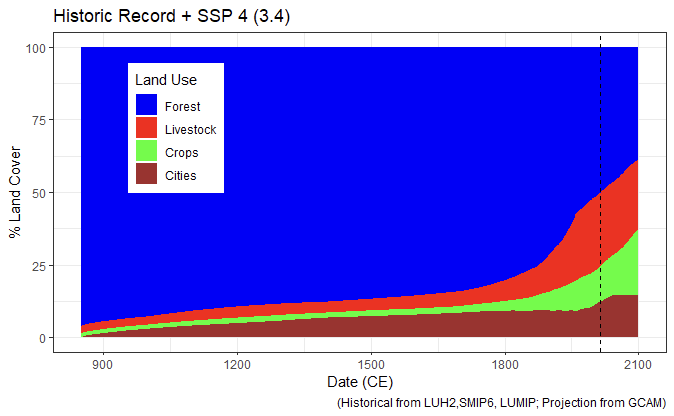
IV. Regional Deforestation
a. Amazon Rainforest
The Amazon Rainforest is the largest rainforest on Earth, but constitutes one of the greatest deforested areas, with some of the greatest climate and ecological consequences. Through the water transferring capacity of its trees, the Amazon plays an important role in driving global atmospheric circulation, and it hosts around a quarter of the world’s land species, including jaguars, sloths, spider monkeys, and macaws.. The Amazon has lost about a quarter of its overall extent in just a few hundred years (Figs. 4, 5).
b. Congo Basin Rainforest
Situated in central Africa, the Congo Basin is the second largest rainforest on Earth, and is home to iconic African wildlife species such as elephants, gorillas, chimpanzees, and lions. The Congo Basin has also experienced deforestation, particularly in the last century, to make way primarily for small-scale agriculture. Today, about 15% of the Congo Basin has been deforested.

SSP1 for the Congo Basin projects a similar forecast to that of the Amazon – significantly reduced deforestation, maintaining about 75% of the original forest area. However, SSP4 for the Congo shows a dramatic difference from the Amazon, with exacerbated deforestation over the next century leaving only 15% of the forests original area by 2100. This is largely due to different economic factors at play between the two continents – South America, having benefitted from the economic gains of, in part, deforestation of the Amazon over the past several centuries, may be able to afford to focus on reforestation and the economic advantages of maintained ecosystem services.
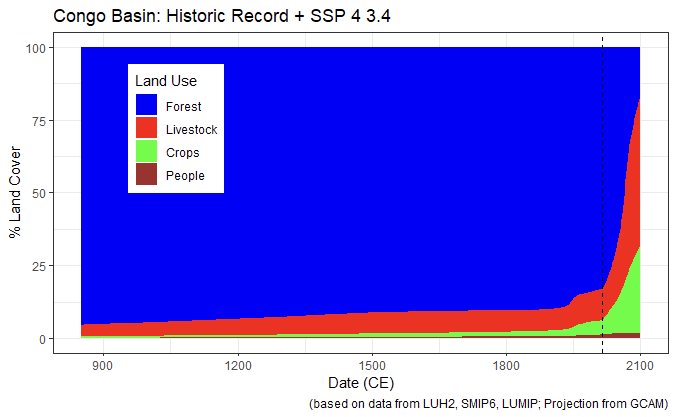

Africa, on the other hand, has not benefitted from as much economic development from natural resource extraction, making continued deforestation to generate cropland and livestock more economically advantageous. Furthermore, projected increases in human population in Africa, and assumed “development” of agriculture there from small-scale to globally-competitive large-scale farming, incentivizes deforestation for economic and social purposes under SSP4.
V. Imagining a Reforested Future
While no SSP projections show globally-significant reforestation, such dramatic increase in forest cover and wildlife habitat would certainly be advantageous for climate and environmental goals, and could be achieved with synergistic benefits to human well-being. Thus, we forced a model projection to simulate how land use changes could restore forests to their geographic extent of the 1980’s over the coming century (Fig. 8). While complicated by a number of social and environmental factors, one study found that global reforestation of 500 million hectares could sequester around 8% of global CO2 emissions per year (Houghton et al., 2015). Thus, reforesting to 1980 levels would restore about 600 million hectares, offsetting around 10% global CO2 emissions per year.
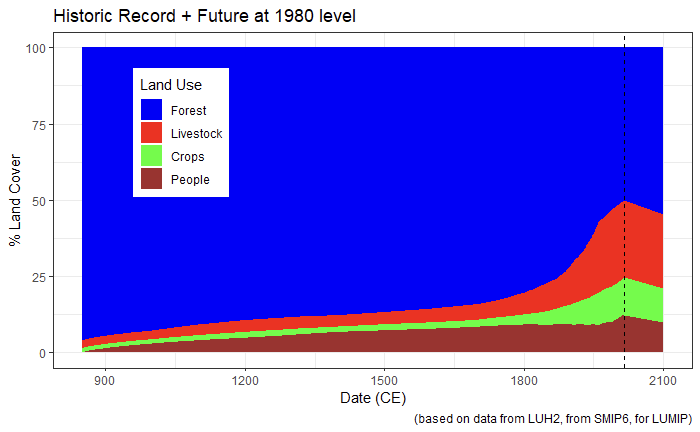
VI. Win-Win Scenarios?
These historical and future data highlight the complexity of the climate change problem – it is as much a social, cultural, and local issue as it is a global problem. Solving climate change and deforestation will require considerations of local economies, cultures, and histories, in addition to wildlife species, local climate, and regional versus global impacts. While no SSPs project globally significant reforestation, certainly reducing deforestation, or reforesting in certain locations, will be critical to protecting and restoring wildlife habitats, ecosystems, watersheds, and climate services that forests provide.
References:
Arora, V.K. and Montenegro, A. 2011. Small temperature benefits provided by realistic afforestation efforts. Nature Geoscience.
Bradshaw, C.J.A., Sodhi, N.S., Peh, K.S-H., and Brook, B.W. 2007. Global evidence that deforestation amplifies flood risk and severity in the developing world. Global Change Biology.
Dass, P., Houlton, B.Z., Wang, Y., and Warlind., D. 2018. Grasslands may be more reliable carbon sinks than forests in California. Environmental Research Letters.
De Sy, V., et al. 2019. Tropical deforestation drivers and associated carbon emission factors derived from remote sensing data. Environmental Research Letters.
Houghton, R.A., Bryers, B., and Nassikas, A.A. 2015. A role for tropical forests in stabilizing atmospheric CO2. Nature Climate Change.
Malhi, Y. et al. 2008. Climate change, deforestation, and the fate of the Amazon. Science.
Newton, P and Benzeev, R. 2018. The role of zero-deforestation commitments in protecting and enhancing rural livelihoods. Current Opinion in Environmental Sustainability.
Shukla, J., Nobre, C., and Sellers, P. 1990. Amazon deforestation and climate change. Science.
Wolff, N.H., Masuda, Y.J., Meijaard, E., Wells,, J.A., and Game, E.T. 2018. Impacts of tropical deforestation on local temperature and human well-being perceptions. Global Environmental Change.
Action Ideas and Resources
- Coming Soon!
- Learn more about what you can do with on the ClimateMusic action page.
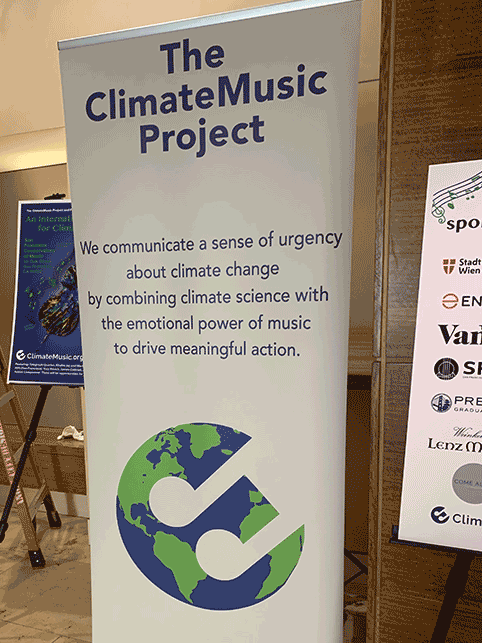
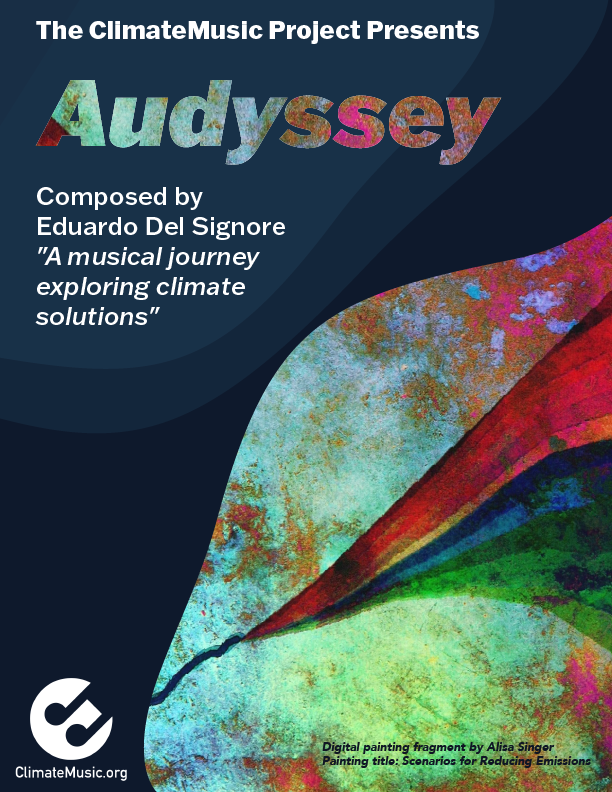
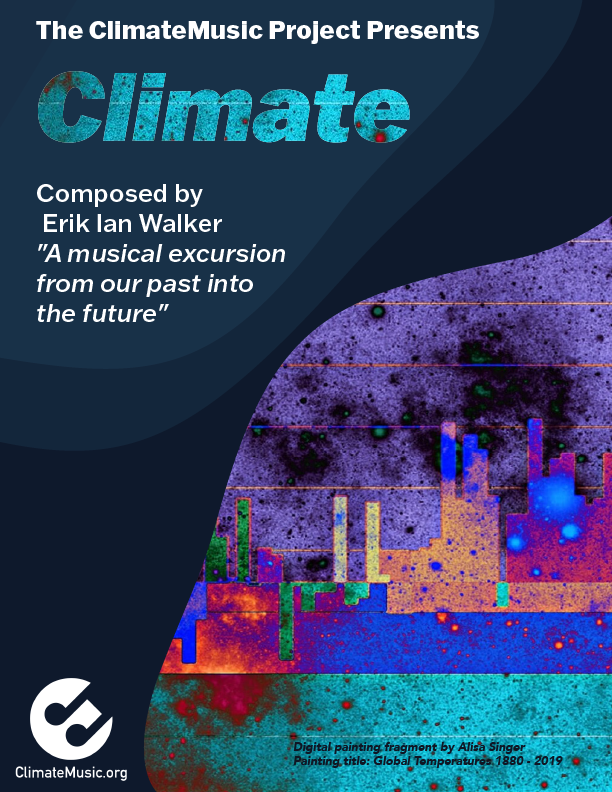
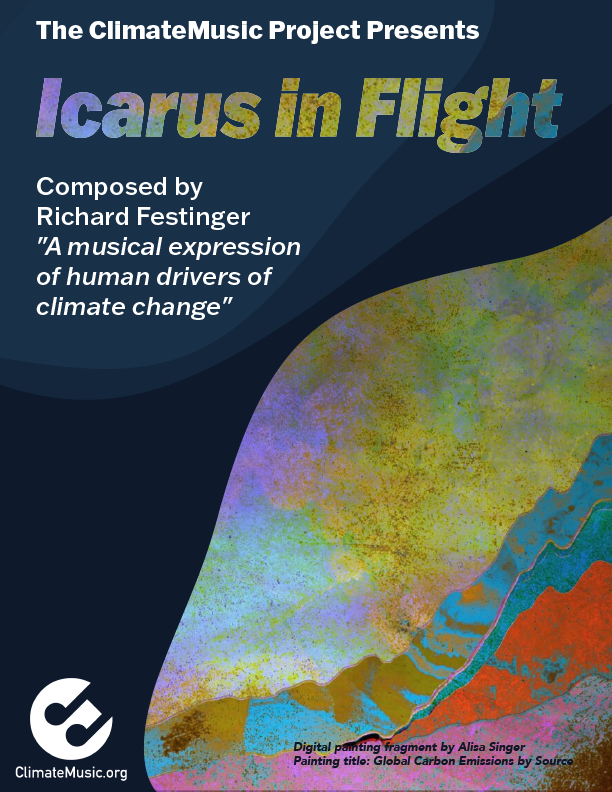
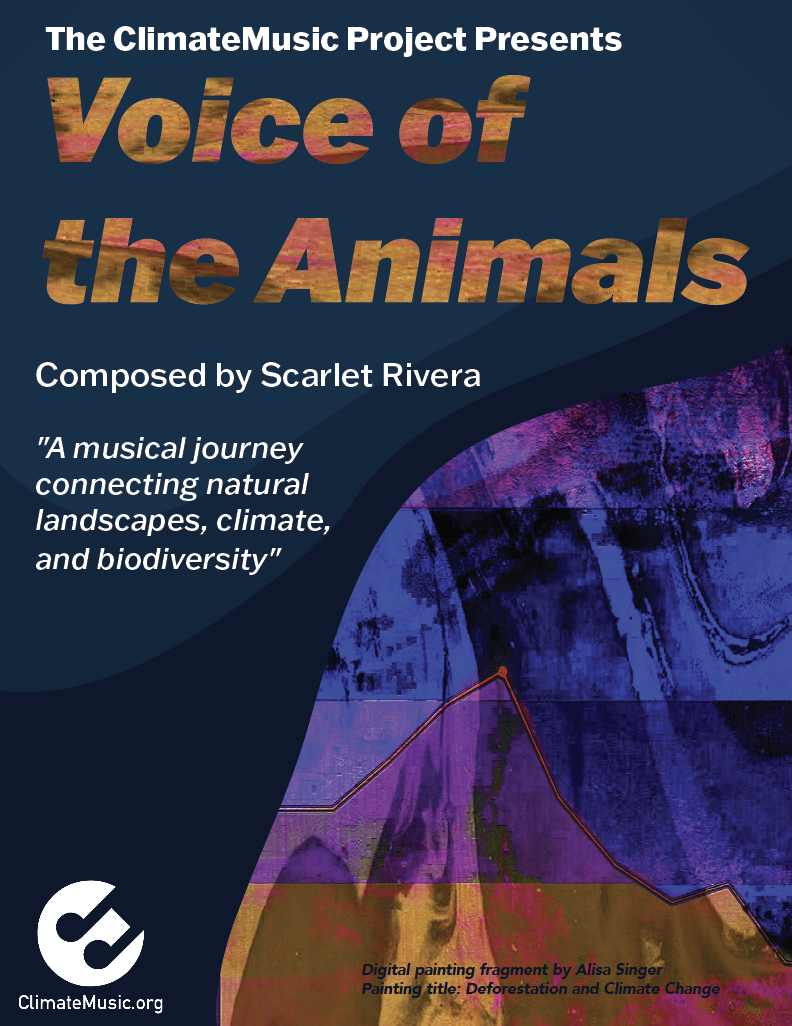
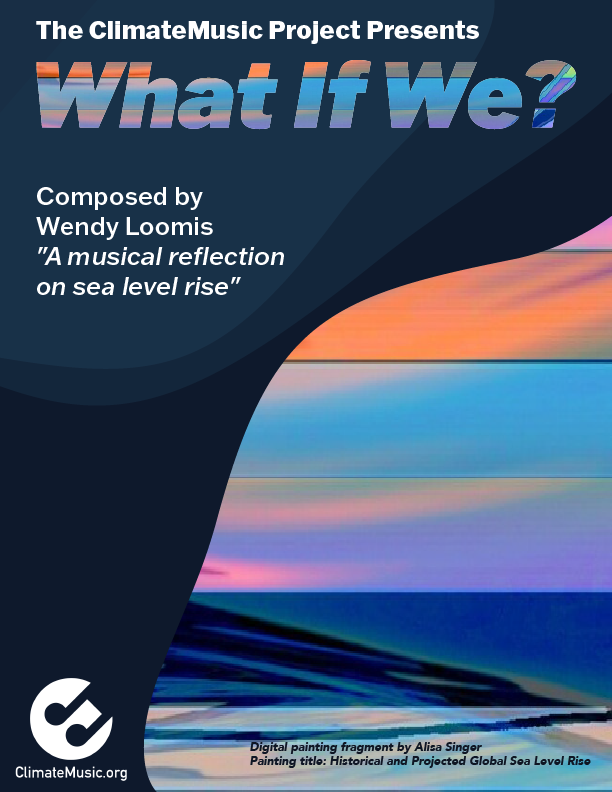